Center for Quantum Science & Technology
Research
A complex communication network requires communication systems to be able to transfer intrinsic/physical information from one or more sources to desired destinations. Accordingly, as the era of quantum information approaches, there is an increasing need to develop communication systems compatible with quantum information technologies. Quantum communication systems use the principles of quantum mechanics, such as entanglement, superposition, time evolution, and measurement, to provide new capabilities for information networks. These quantum approaches improve the security and performance of classical communication systems and, more importantly, enable the transmission of quantum information. Two essential factors promise the achievement of quantum communication systems in the near future. First of all, light can provide the required quantum characteristics to develop and engineer quantum communication systems. Second, due to the development of fiber optic networks, high-rate information transfer is available via optical communication signals.
The most important aspects of research in the field of quantum communication systems are:
- Enhancing classical communication systems: Signals and transmission media of typical communication systems are usually modeled using classical or semi-classical physics. However, quantum mechanics renders a more accurate description of communication signals and channels, where the transmitted and received signals are quantum states of light. Designing the optimal receiver according to the statistical properties of the received communication signals plays a significant role in the performance of communication systems. In 1976, Hellstrom developed the fundamental concepts of quantum communication for classical information transfer in terms of quantum detection theory and showed that considering the quantum nature of signals in receiver design would lead to better performance than the classical optimal receiver. Since then, this field has become an active research area.
- Ultimate secure communications: Conventionally, secure communications such as public-key cryptography are typically based on computationally hard mathematical problems. The development of quantum computers in the coming years will jeopardize the classical schemes for secure communication. While quantum technologies threaten classical information security methods, they enhance security by offering an alternative. Intrinsic quantum information security resulting from the fundamental principles of quantum mechanics, such as the no-cloning theorem and Heisenberg's uncertainty principle, makes quantum key distribution (QKD) possible. This method enables communication systems to provide secure communication for users. Therefore, since the influential work of Charles Bennett and Gilles Brassard in 1984 on QKD, this research area has attracted the attention of researchers. Due to the crucial role of security in information technology, this area has become one of the main drivers of the commercialization of quantum communication technologies.
- Transferring quantum information: Classical distributed computing systems are an essential application formed in the context of current communication systems. Therefore, it can be expected that the distributed processing of quantum information will be considered a key element in the quantum communication system horizon. The development of quantum computing and processing eventually requires transmitting quantum information in such distributed systems. Therefore, modern quantum communication systems must be able to share information according to the needs of distributed quantum systems. Quantum communication systems can provide the basis for the development of future distributed quantum systems by using methods such as quantum transmission, quantum coding, and distributed entanglement.
For example:
- Wavelength division multiplexing (WDM) is one of the principal methodologies in conventional and global optical fiber communication networks where each pair of users uses a particular wavelength to transmit information. Our work on all-quantum multi-access WDM communication systems (QWDM) studies the evolution of generic quantum signals among WDM devices in a systematic and unified approach (see Fig 1).
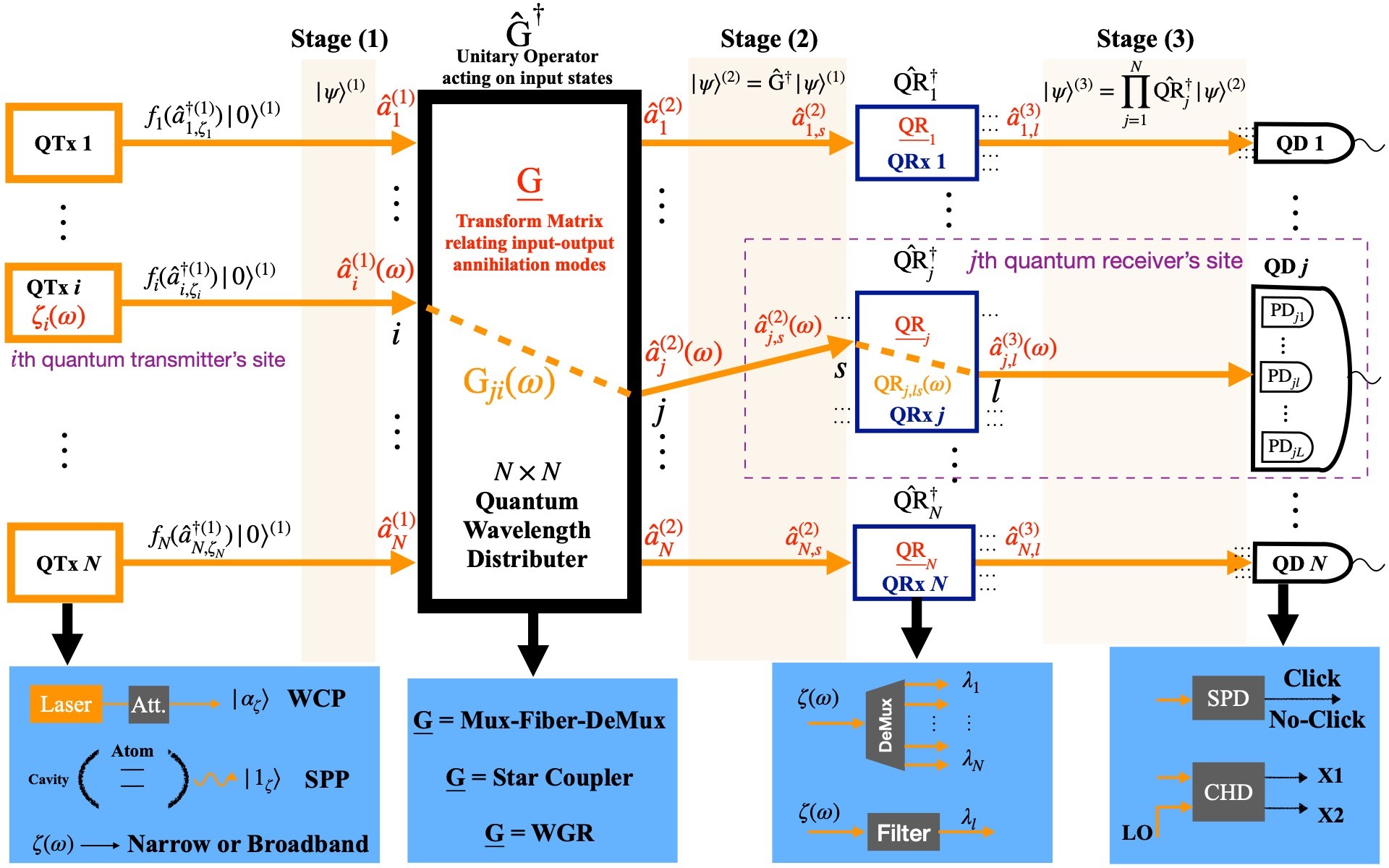
- In code-division multiple-access (CDMA) techniques, communication between a pair of users requires that each sender encodes or imprints upon its output information bits a pseudorandom binary sequence (barcode) that corresponds to the intended user’s signature sequence. CDMA gives specific advantages such as high spectral efficiency, distributivity, data privacy, and asynchronous transmission amongst the users in the network. We introduced novel quantum CDMA (QCDMA) networks based on spectrally encoding/decoding input quantum light pulses (see Fig 2). Ref [1] introduces the mathematical model of quantum spectral encoding and decoding and broadcasting star coupler that can treat any input pure quantum state of light, such as Glauber state, number state, and squeezed state.
[1] M. Rezai and J. A. Salehi, Quantum CDMA communica- tion systems, IEEE Transactions on Information Theory 67, 5526 (2021).
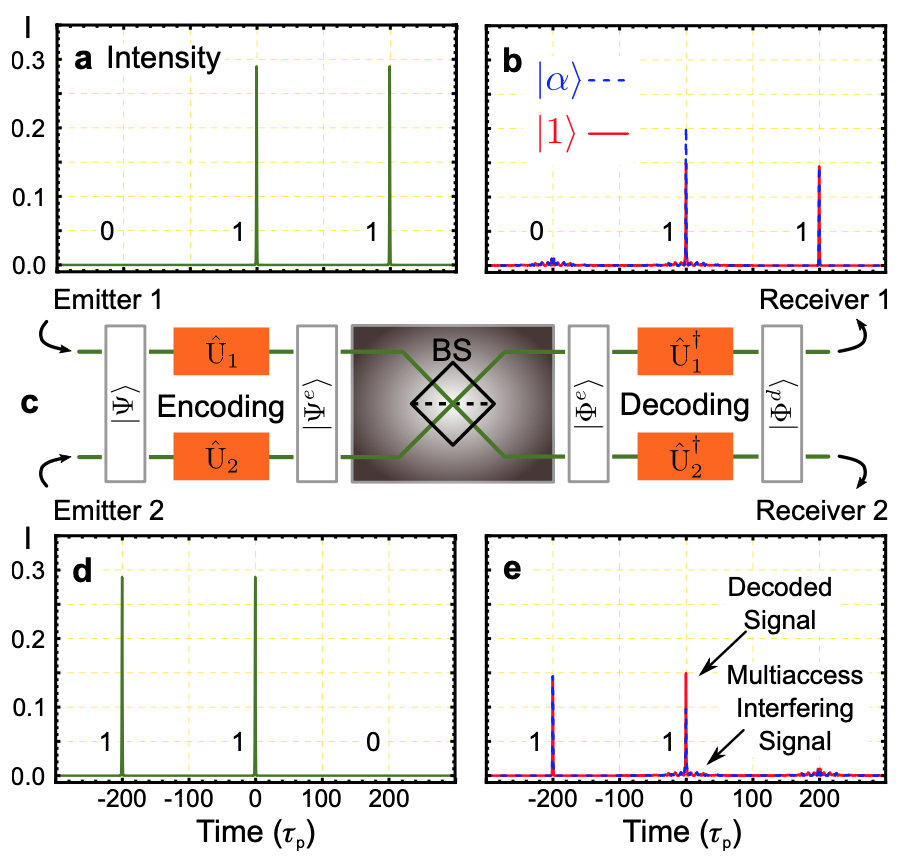
Photon-based quantum computation, due to the unique features of photon qubits, such as high coherence time and their non-substitutional role in quantum information-carrying, enjoys special attention. For example, quantum Fourier optics developed in SQIST takes advantage of the vast capacity of photon wavefront (image) for quantum information encoding and processing and linear optical quantum computing (see Fig 1).
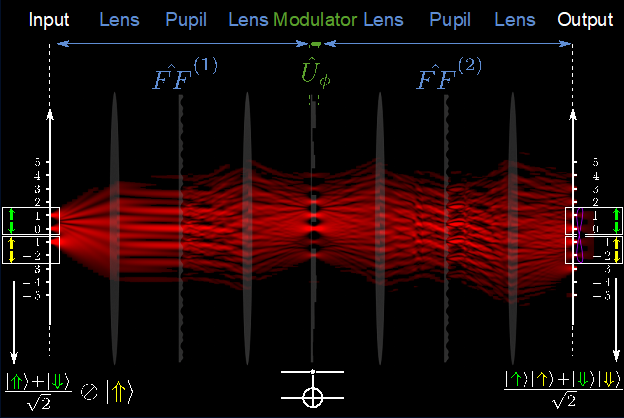